How A Simple Formula Can Help You Find Aliens
Since the beginning of human civilization, we have looked up at the
stars and wondered in amazement. The vastness of the universe was
unknown to early humans, but in recent decades and centuries, we have
begun to realize just how incredibly large the universe truly is. With
hundreds of billions of stars in our galaxy alone, and with hundreds of
billions of galaxies in the observable universe, a normal question was
bound to arise… Is anyone else out there?

This has fascinated everyone from conspiracy theorists and kings to
modern astronomers and Hollywood producers, but back in 1961, a man
named Frank Drake came up with an equation that would measure the
likelihood of making contact with intelligent life in our galaxy. That
is the essential goal of the Drake Equation, and while it has been hotly
debated for decades, it remains one of the most concise and trusted
methods of estimating an answer to that eternal question:
Are we alone in the universe?
The Drake Equation
When Frank Drake first came up with the idea for the Drake equation,
he was actually trying to generate a conversation among a “meeting of
the minds” regarding the search for extraterrestrial life (SETI).
However, the simple and inclusive design of his equation has become the
stuff of legend. Before we go into the details of the equation, let’s
look at it in simple terms.

The Drake Equation (Photo Credit: meletver / Fotolia)
So, the Drake Equation is:

However, it would be a bit more insightful to know what each of those variables meant. So, without further ado…
N = the number of possible civilizations in the galaxy that could communicate with us
R* = average rate of star formation in the Milky Way
fp = the fraction of those stars that possess planets
ne = the number of planets that could support life around each of those stars with planets
fl = the fraction of planets that could support life that actually do develop life
fi = the fraction of planets with life that develop intelligent life
fc = the fraction of intelligent civilizations that attempt to send communication out into space to be detected
L = the length of time that signal would be sent out into the universe (duration of civilization)

The Drake Equation (Photo Credit: noeticscience.co.uk)
An Answer to the Drake Equation?
While it seems like a simple enough equation, the problem doesn’t
like with generating a final answer (N), but rather in determining what
other variables should be included. The first three variables (average
star formation rate, planet-possessing stars, and potential to support
life on those planet) are
relatively easy to determine.
R* – By looking at our own nearby galactic
neighborhood and the residual gas clouds from star formation, we can
safely calculate that the present rate of star formation is currently
about 7 stars per year. This number was far greater in the past, but in
the 21st century, that’s a safe bet.
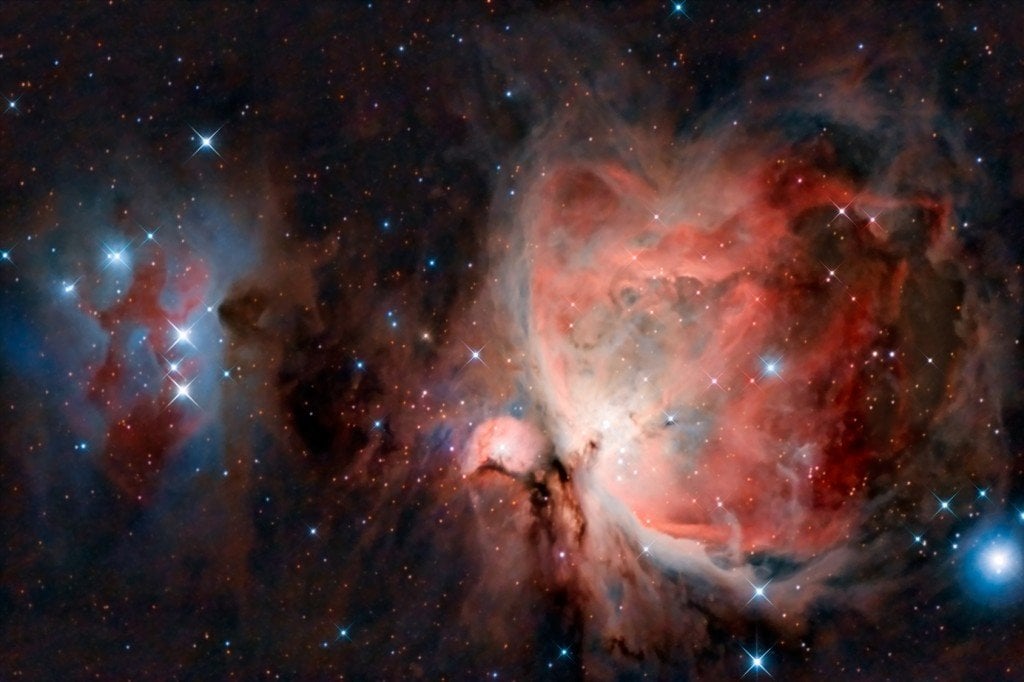
The Orion Nebula, one of our nearest star nurseries (Photo Credit: peresanz / Fotolia)
fp – The fraction of those stars with planets is
often assumed to be 1, given that many modern astronomers believe that
every star has the capacity to retain orbiting planets, making stars
without planets an exception, rather than a rule. However, some other
figures place that number at only .4 (4 out of 10 stars will possess
planets). This is where the significant differences in variables begin,
but it gets even more intense.
ne – The number of planets that
could
support life around each of those stars is largely derived from our own
solar system, where Earth is definitely habitable, but we have yet to
determine the habitability of various other moons and planets.
Therefore, the number ranges from .5 to 2 in most calculations of the
Drake Equation.
Those are the easy ones… and there is far more debate on the
last 4 variables, as much of it is conjecture, based on belief, rather
than scientific fact or direct observable experience.
fl – The fraction of planets that
do
develop life is hotly debated and cannot be directly tested, as we
haven’t traveled to other exoplanets where the “stuff of life” has been
detected. We know that life could develop, but not whether it will. Some
say life is inevitable, making this variable equal to 1, while others
say it is 1/100, or even smaller!
fi – The fraction of planets with life that
develop intelligent life is similarly up for grabs. Many argue that
intelligent life is inevitable, using Earth as an example (humans
evolved from every other form of life, and eventually became
intelligent), while critics say that it was a 1 in a billion chance, as
there have been hundreds of millions of non-intelligent species in the
history of the planet.
fc – The fraction of those intelligent
civilizations that send communication signals suggests a highly
technologically evolved culture with the desire to reach out to the
stars. This could also be largely conjectural, but a 1/100 estimate was
made by Drake, although others say it is a 100% chance, if given enough
time.
L – Finally, we come to length of time the signal would be generated.
For example, perhaps a civilization rose to prominence 10 million years
ago, broadcast the signal, but then destroyed itself before it could
connect with us. This is the variable with the most flexibility, from a
few hundreds years up to billions (some suggest that eventually, a
civilization will discover how to survive in perpetuity, meaning the
signal transmission time would be massive.

Maybe they’re just getting a busy signal (Photo Credit: Alexander Pokusay / Fotolia)
NOW….in 1961, Drake estimated with a rather simple range of values, and came up with two values, a minimum and maximum.
The minimum estimation of possible communicative civilizations in our
galaxy is 20, while the maximum is roughly 50,000,000. Frank Drake
himself believes that the number lies somewhere between 1,000 and
100,000,000.
The Modern Take on Drake
While the debate over extraterrestrial life will never be resolved
until we actually find some, we have improved our ability to calculate
the variables in the equation. Unfortunately, this hasn’t done much in
terms of shrinking the possible range. In fact, our precision has only
expanded the range, and current estimates range from 2 to 280,000,000.
The original purpose of the Drake equation, we must remember, was not
to determine an accurate number of communicating, intelligent
civilizations in our galaxy; it was to stimulate thought in this area,
and hopefully guide people to look into the variables and expand the
scope of their research. To that end, Frank Drake has been extremely
successful. With every successive venture into the cosmos, we get closer
to finding life (or not), but with hundreds of billions of stars out
there, we’ll likely never know the exact number. However, in terms of
extraterrestrial life, I’ll leave it to Fox Mulder….