From the books on our shelves to the newspapers, magazines, and documents in our hands, we interact quite a bit with ink on paper. Obviously, we don’t do this nearly as much as we used to, since the rise of cell phone technology, but there is still something quite nostalgic about the printed word, rather than the digital one.
Pressing ink into paper has been going on for nearly 2,000 years, and it has been one of the most important elements of sharing knowledge and information for cultures around the globe. However, ink is a strangely resilient material, and with a few notable exceptions (like cheaply printed newspapers), the ink always sticks to the page. You’ve read countless ink-pressed pages in your life, but have you ever wondered what keeps the ink on the page?
The Science of Paper and Ink
The majority of paper is manufactured in a similar way at the basic level – an even distribution of fibers (made of cellulose) that have small depressions between them, often called pores. These pores are microscopic in nature, and essentially “suck in” ink through capillary action.
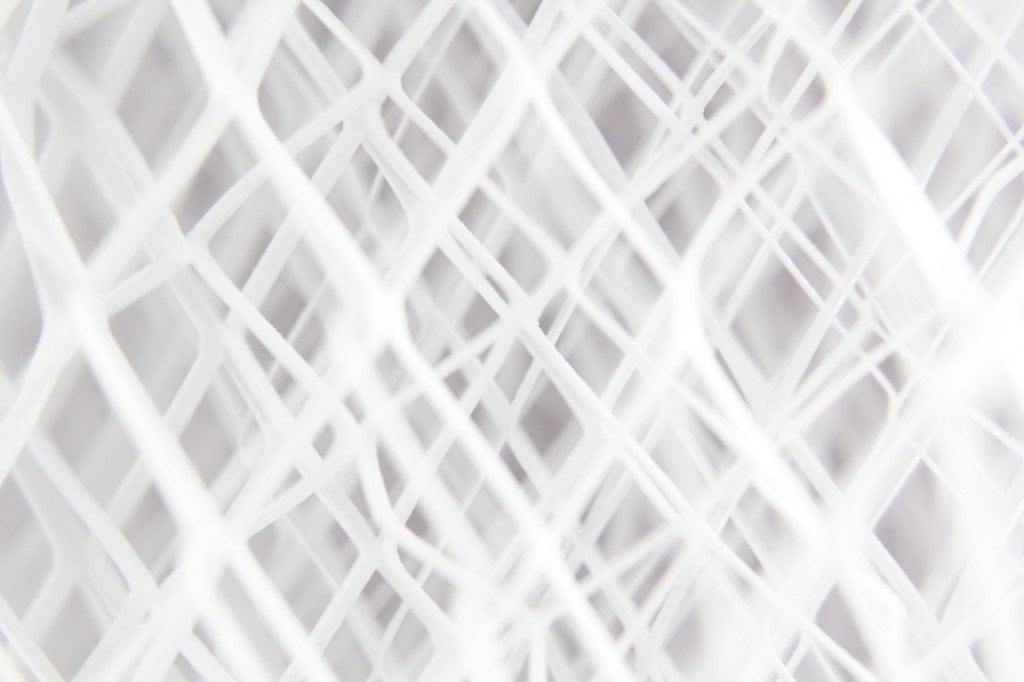
Paper Fiber Super-Zoom (Photo Credit: tks2 / Fotolia)
For those of you who don’t know, capillary action is the unusual ability of liquids to move into narrow spaces against or without the assistance of gravity. This phenomenon is actually seen all over, from the sand getting wet at the beach to the way paint moves up the small tubes and hairs of a paintbrush. This occurs as a result of intermolecular forces that are acting on both the exterior surface and the liquid surface.
A combination of surface tension of the liquid, in this case ink, and the adhesion properties of the paper fibers, causes the ink to move down into the pores of the paper, where it will remain to dry. Papers do come in a range of different “porosity”, and you’ll notice that some glossy papers for photographic printing, have no porosity at all, completely enclosing the fibers and preventing ink from soaking in and drying in the same way. Variations in the porosity affect the amount of time ink takes to dry.
Highly absorbent paper actually takes longer to dry, because as more is absorbed, it becomes harder for oxygen to reach it. Therefore, slightly glossy paper (but still porous) will dry faster because there is more ink on the surface that is being exposed to oxygen and drying.
Why Can’t I Erase a Pen? I Can Definitely Erase Pencils…
Pencils are primarily composed of graphite, and when you draw the pencil across the paper, some graphite particles get stuck on the paper fibers, leaving behind the marks and letters you just wrote. When a traditional rubber eraser is used to eliminate these graphite marks, they are being rubber over the surface to create friction, which heats up the rubber molecules, making them sticky. These sticky molecules then pluck out those graphite particles that are on the very top layers of the paper fibers. Suddenly, poof! No more graphite lines.
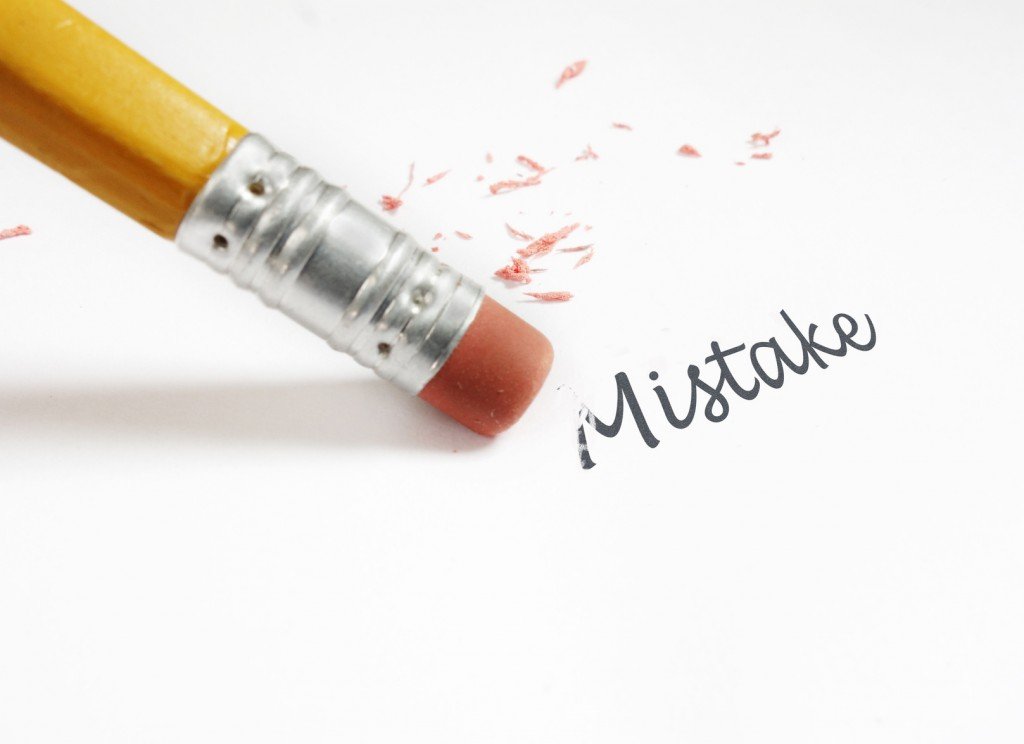
The Reason We Use Pencils! (Photo Credit: zimmytws / Fotolia)
However, pens are different, as the dyes used in ink pens is a liquid, and seeps deep into the fibers of the paper. When you try to erase marks from a pen after they have dried, the rubber is unable to separate the intermolecular forces, and you would have to physically scratch down to the level of the dried dyes and eliminate the text that way. For many types of paper, however, which have very high porosity, the ink may bleed nearly all the way through, making it impossible to erase with a traditional erase without destroying the paper itself!
Does the Ink Play a Role?
The type of paper used is not the only reason why ink can be absorbed and dried on a piece of paper; the ink itself also plays a part. There are many different types of ink today, but the primarily used type in printing books and newspapers is carbon black pigment, in addition to various surfactants, resins, waxes, lubricants, and drying agents. This pigment is mixed in with what many call a varnish (or vehicle), which moves the color component to the paper. At that point, the varnish is also responsible for helping the ink harden (or dry).
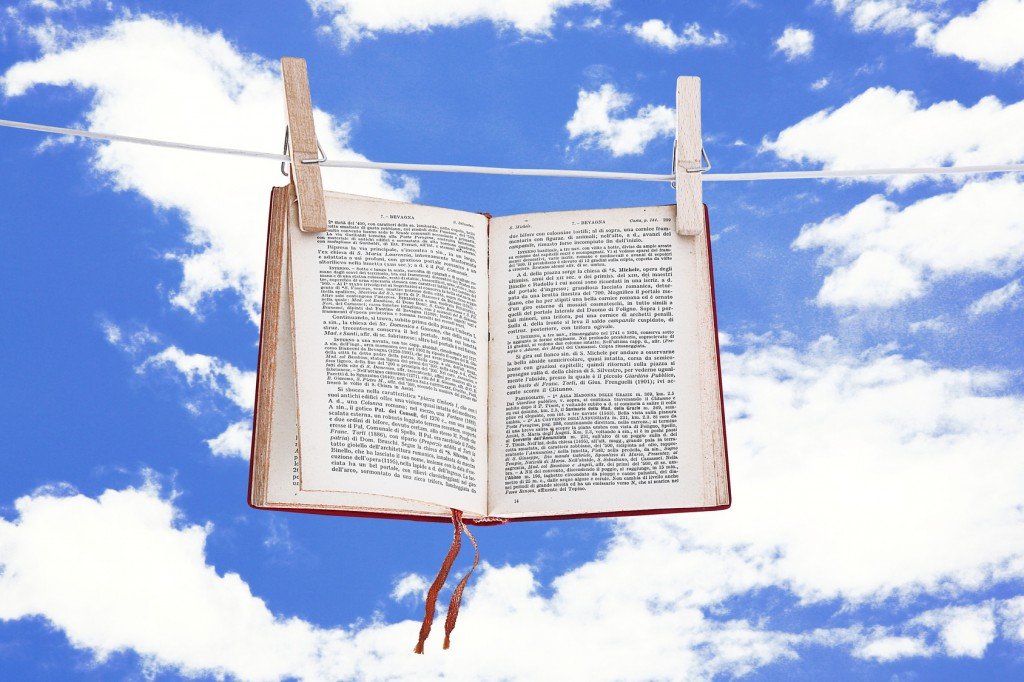
“Drying Ink” (Photo Credit: Giuseppe Porzani / Fotolia)
This varnish is composed of resins, mineral oil, and vegetable oil, which work in tandem to promote drying. The mineral oils must be absorbed, but the resins and vegetable oils will oxidize when spread out in thin layers; this promotes drying on the surface, even if some of the ink below is not completely hardened. Choosing ink with a well designed mix of drying agents and surfactants keeps ink in place on the paper for years to come, unless it gets wet, of course!
What’s With the Ink On Our Fingers?
Cheaply printed newspaper use high absorbency paper and mineral oil-based inks, which are less expensive. Those types of oils dry much better when the paper is heated, but newspapers are printed extremely quickly – thousands of pages per minute – so there is no time to heat the paper and ensure proper drying before the papers are shipped out to news stands all over the country.
Some of the ink on a freshly printed newspaper will remain on the inner fibers of the paper, but in those great big stacks of newspapers, there isn’t a whole lot of oxygen available to dry the remainder of the ink. Therefore, as you’re flipping through the paper, the skin on your fingers experiences some absorption of the ink (as described at the top of the article, with the capillary effect).
If you don’t mind having slightly smudged fingers, then reading the newspaper is probably still a beloved pastime, but for those who have no tolerance for ink that doesn’t stay where it belongs, perhaps you’d be better off reading your news on your smartphone screen!
source: www.scienceabc.com